
First observation of fast decomposition process of high temperature and supercritical water by ionizing radiation
Jan. 28, 2010
1.Background
Due to low CO2 emissions, nuclear power has recently been reevaluated as a sustainable and environmentally benign energy source to avoid global warming.. In developed countries there is renewed interest in further construction of nuclear power plants and many developing countries are planning to introduce nuclear energy.
It is well known that coolant water in nuclear reactors receives high radiation doses under a mixed radiation field of gamma rays and fast neutrons at high temperature and pressure. Radiation controls the chemical condition of the coolant and precise prediction of radiolysis effects is essential to avoid detrimental radiation-induced corrosion processes such as stress corrosion cracking (SCC). Because of the strong radiation field under the high temperature and pressure, it is impossible to do direct measurements inside the reactor. Thus, computer simulations are usually performed for the evaluation of coolant water radiolysis at various places in the reactor. However, although some necessary data sets for these simulations have been accumulated, most of the experiments are limited at 230 コC due to the difficulties associated with performing pulse radiolysis experiments using very short electron pulses and absorption spectroscopy methods at high temperature and pressure. Extrapolation methods have been applied to simulation operating temperatures around 300 コC. Consequently, more reliable data is necessary in order to characterize the corrosion environment inside light water reactors (LWRs) and especially one of the next generation reactors ・supercritical water-cooled reactors (SCWR).. As shown in Figure 1, when temperature T > 374 コC and pressure P > 22.1 MPa, water enters a special region called the supercritical state, and the characteristics of supercritical water are quite different from those of solid, liquid and gaseous water. Since most reactions are accelerated with increasing temperature, it is essential to develop a measuring system to study them above 230 コC. Based on an international collaboration of Japan Atomic Energy Agency, the University of Tokyo and University of Paris-Sud (France), much effort has been made in this direction using the high performance picosecond accelerator at the University of Tokyo, as shown in Figure 2. For the first time, we have succeeded in measuring the kinetic behaviors of the hydrated electron ・one of the main products of water radiolysis, within a time range from 60 picoseconds (6ラ10-11 s) to 6 nanoseconds (6ラ10-9 s), from room temperature to 400 コC. The increasing fast decay rate with increasing temperature has been observed.
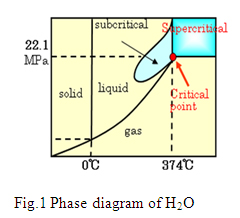
2.Results and discussion
In order to measure early processes of radiation-induced reactions in high temperature/high pressure water, a new setup was constructed.. Usually the radiation induced reactions are very fast. To monitor a reaction from the very beginning of irradiation to the end, it is essential to have a detection technique with high time resolution. Currently, although time resolution of a few picoseconds (1 picosecond = 10-12 s) has been achieved by the pulse radiolysis method, it was only available for samples at room temperature. The application of this technique to samples at elevated temperatures or under supercritical conditions resulted in many problems with regard to spectroscopic measurements. The time resolution remained at nanosecond (1 nanosecond = 10-9 s). Therefore, in present study a new pulse radiolysis system was developed to overcome those problems.
In order to observe above ultrafast reactions, it is essential to resolve the following two problems:
(1)Shortening of pulse width and strengthening of the electron beam
First, it is necessary to have a very short radiation pulse. The electron beam used to irradiate the samples must trigger the reaction instantaneously. Besides this, since a larger chemical change due to irradiation will be expected in an easier detection, it is essential to have higher beam intensity. Further, samples at elevated temperatures must be kept in a thick chambered containers, and the electron beam must be able to penetrate this chamber wall. Therefore, a Linac accelerator with a femtosecond laser-driven photocathode, able to generate short pulse width and intense electron beam was used. An electron beam with an energy of ~22MeV and a charge of 2.5・.8 nC was focused to a diameter of 3 mm. Then it was possible to irradiate the sample inside the vessel with an absorbed dose of 13・5 Gy/pulse. In the present work, the intensity of the electron beam was 4・ times higher than has been used before.
(2)Measurement of radiation-induced chemical reactions with ultra-high time resolution
To study the radiation induced chemical reactions with the high intensity and short electron pulse discussed above, it is necessary to precisely measure the transient species produced in the sample solutions. Taking advantage of the optical absorption of the transient species, we were able to analyze them by measuring a small change in the light passing through the sample. Using femtosecond laser as the analyzing light, it is possible to have a 壮nap-shot・of the sample at a given time after irradiation. So we were able to monitor the reactions dynamically through the difference in the absorptions obtained at the times of irradiation and analysis. Although this method has been used previously for measurements at room temperature, there were problems when applied to the samples at high temperature and high pressure. Because the analyzing light was drastically scattered by the flow of the sample through the optical cell at high temperature and high pressure, it was rather difficult to conduct a precise measurement. In order to resolve this problem, we used two laser pulses with very short time interval (about 11 ns) as reference and analyzing lights, and adjusted the timing of electron beam just between these two laser pulses (i.e. before and after irradiation). Because of the very short interval of these two pulses, we were able to avoid light perturbation due to fluctuation in the sample flow. In addition, a new and unique program for machine controlling, data acquisition and analyzing has been developed. As a result, we have achieved a marked improvement of the precision from ~2% to 0.5%.
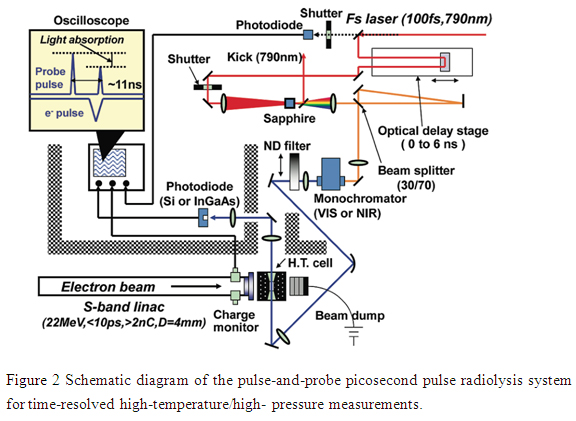
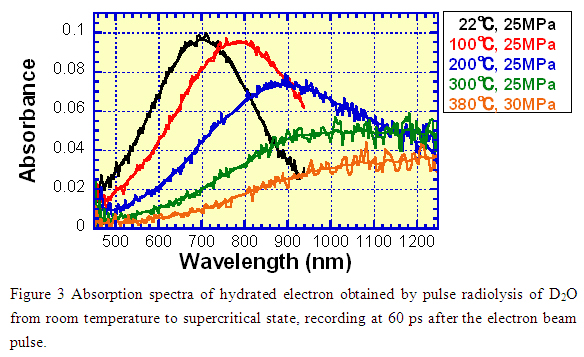
Accordingly, because of an improvement of 4-5 times in both beam intensity (signal intensity) and measurement precision as compared to previous work, we succeeded in developing a new pulse radiolysis system capable of tracing radiation- induced reactions with picosecond time resolution for high temperature and supercritical water.
As an example, the experimental result using this system is shown in Figure 3, which exhibits the absorption spectra of the hydrated electron recorded at 60 ps after the electron beam pulse. At room temperature, there痴 a strong absorption band with a maximum at about 700 nm. With increasing temperature, the spectrum shifts to infra-red region and the absorbance decreases. We were able to record the optical absorption spectrum under supercritical conditions (380コC/30 MPa) where the absorbance became very small.
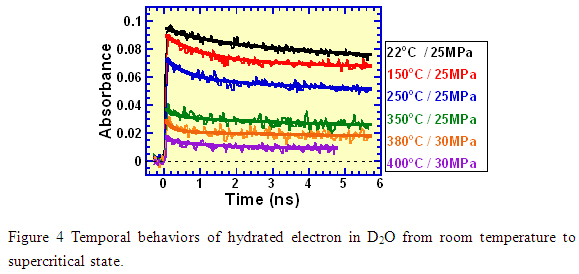
Meanwhile, we also succeeded in measuring the decay kinetics of hydrated electron from picoseconds to nanoseconds, as shown in Figure 4. At room temperature, it is well known that after formation at very short times (<1 ps) after irradiation, the hydrated electron slowly decays till ~100 ns due to spur reactions (complicated reactions between various transient species of water decomposition). For the temporal behaviors at elevated temperatures, there were only a few reports on computer simulations and even for these the temperature was limited to 300 コC. In addition, these reports were different from each other. A model for temperature higher than 300 コC has not been constructed yet. Therefore, the first observation of the decay kinetics of the hydrated electron in the present work is expected to make a significant contribution to the understanding of water radiolysis at elevated temperatures and supercritical conditions.
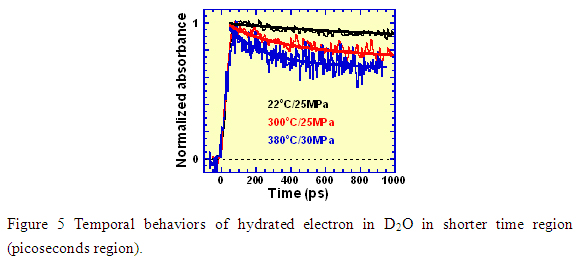
3.Future plans
Figure 5 shows a comparison of the temporal behavior of hydrated electron in the picosecond region (< 1 ns), from room temperature (22 コC), to high temperature (300 コC) and the supercritical state (380 コC). In each case the signal built up quickly at time zero (immediately after irradiation); however, the decay was clearly different. It is well known that the transient species of water decomposition include ・/sup>OH, ・/sup>H, H+, H2 and H2O2, in addition to the hydrated electron. At room temperature, very complicated reactions occur among these transients immediately after irradiation to 1 µs,. For reactions at elevated temperatures a conventional time resolution of 10 ns was not sufficient to do the measurements. However, with picosecond pulse radiolysis, we were able to demonstrate that the reactions at elevated temperature (300 コC) became faster than at room temperature and much more drastic under supercritical conditions where 30% of hydrated electron disappeared within about 600 ps, as shown in Figure 5. This implied that the hydrated electron should react with some substances. Possible reactions of the hydrated electron with important water decomposition products e-aq, OH, H+ (eaq- + ・/sup>OH → OH-; eaq- + H+ → H・/sup>; eaq- + eaq- + 2H2O → H2 + 2OH-) can be listed; however, it is likely that the reaction with H+ will be dominant.. The role of this reaction will be elucidated by further experiments.
Furthermore, the effects of pressure on the temporal behavior of the hydrated electron under supercritical conditions (400 コC) were also investigated. As mentioned above, the water density is greatly dependent on pressure in the supercritical region. For example, in Figure 6, at 400 コC, the water density at 40 MPa is about 3 times higher than at 25 MPa. According to the measurements of the hydrated electron at very different water densities, its yield (G value) changed greatly with density. It is noted that not only density but also dielectric constant, ionic product, viscosity, solubility and many other properties change with temperature and pressure. The main factors affecting water radiolysis will be elucidated in the further experiments.
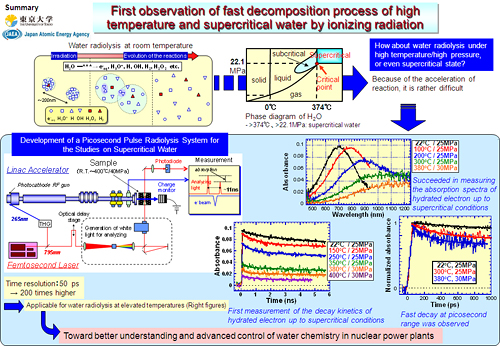
|

Location of environment monitoring posts measuring amount of
radiation. (details)

International link directory of related websites.
|